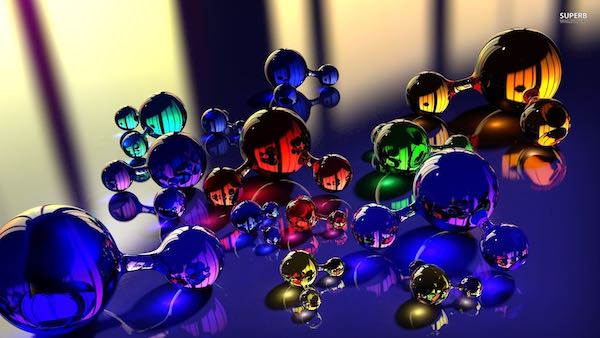
Credit: ビッグアップジャパン; Flickr CC BY-SA 2.0
Glass is an incredible material. So incredible, in fact, that some say current times can arguably be dubbed the Glass Age.
And now, using the power of a supercomputer at the Texas Advanced Computing Center at the University of Texas Austin, a Georgia Tech professor of mechanical engineering is going to save the world—and glass is going to play a central role.
“The way we produce electricity today pollutes the environment,” Georgia Tech mechanical engineering professor Asegun Henry says in a Texas Advanced Computing Center press release. “My research is primarily centered on converting us from a fossil fuel-based infrastructure to a renewable- or solar-based infrastructure so we can rely on renewable forms of energy that don’t pollute the world at all. …I personally see that as saving the world.”
Glass is an integral material when it comes to energy use and renewable energy applications.
According to Henry, more than 10% of U.S. energy use is directly related to glass, considering energy loss through glass windows, energy harvesting capabilities of glass-topped solar cells, and roles for glass materials in nuclear energy storage, next-gen batteries, and much more. I suspect that number could actually be much higher than 10% once you figure all the indirect relations, too.
But despite the ubiquity of uses of glass in today’s (and tomorrow’s) world, we’re still learning about the properties of glass as a material.
For instance, although we have sufficient models to predict how heat conducts through crystalline materials—the phonon gas model (phonons are quasiparticles associated with vibrations in a crystal)—amorphous materials such as glass are more of a mystery. The phonon gas model doesn’t accurately describe how these materials conduct heat.
And because glass and other amorphous materials, including plastics, play such a key role in energy-related applications, a better understanding of heat conductivity through these materials can help design new energy-saving materials and applications.
In developing a more accurate model to predict heat transport through amorphous materials, Henry’s research expertise in atomic simulations gives him a unique perspective.
“I understand different processes in terms of what’s going on at the atomic level,” Henry says in the release. “That allows me to develop insights and opportunities for new ideas that are different from others that are coming from the macroscopic level.”
Those insights have helped Henry and his colleagues develop a new model, using molecular dynamics simulations to more completely encompass the contribution of different vibrations within amorphous materials. The model, called Green–Kubo Modal Analysis (GKMA), more accurately models phonon dynamics.
And using the power of supercomputing, the scientists simulated whether that model can predict how glass’s thermal conductivity increases with temperature.
The team’s analysis simulated the behavior of localized atomic vibrations within glass, measuring whether they contribute to glass’s ability to transfer heat.
Credit: TACCutexas; YouTube
“Unlike crystalline materials, where the vibrations become collective motions that act like sound waves, in amorphous materials, you get a different kinds of vibrations, the majority of which look random, like the underlying structure,” Henry explains in the release. “You even get small, localized vibrations that consist of only dozens of atoms.”
But previous models have ignored these localized vibrations.
“The assumption was that they don’t contribute at all,” Henry adds in the release. “But what was surprising that we found with our new method was that in this specific material, the localized modes contribute substantially.”
The supercomputing results showed that localized modes contributed more than 10% to total thermal conductivity and are the primary reason why glass’s thermal conductivity increases as it heats up.
Henry says, “We’re at the edge of pushing our community to rethink the problem of thermal conductivity and exploit behaviors to achieve properties that were previously thought to be impossible.”
The open-access paper describing the GKMA model, published in Scientific Reports, is “Examining the validity of the phonon gas model in amorphous materials” (DOI: 10.1038/srep37675).