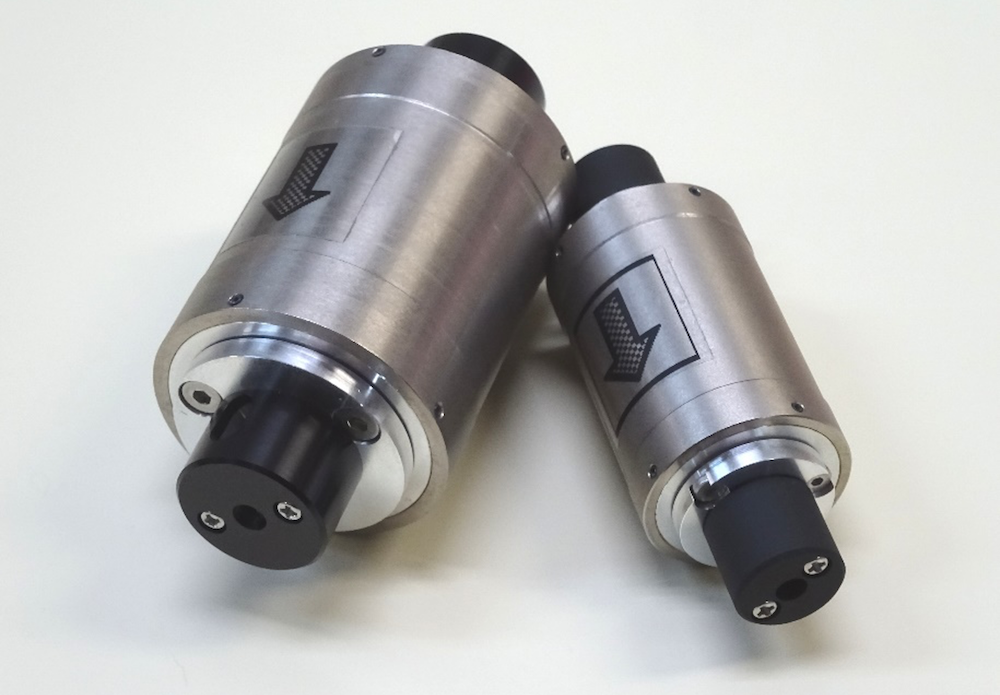
[Image above] Prototype of an optical isolator using terbium yttrium oxide ceramic (right) in comparison with a commercial optical isolator based on terbium gallium garnet single crystal (left). The ceramic optical isolator is smaller because the terbium-based ceramic has a higher Verdet constant, meaning smaller magnets can be used to construct the device. Credit: Akio Ikesue
From the first report of a working laser in 1960 until the mid-1990s, a laser’s gain medium—or the material used to amplify the power of light—was made using a single crystal.
Single crystals work well in optical applications because they have no grain boundaries, unlike polycrystalline materials. However, producing single crystals is a difficult process.
When an optically transparent polycrystalline material with properties close to single crystals was reported in 1995, the impact on laser technology was immense. These polycrystalline materials were easier to produce, and now these “transparent ceramics” serve as the gain medium in many commercial lasers.
A laser’s gain medium is not the only laser component that traditionally relied on a single crystal—single crystals are also key to optical isolators.
Optical isolators: Control the direction of light
An optical isolator, or optical diode, is an optical component that allows transmission of light in only one direction. It typically is used to prevent back-reflected light into an optical oscillator, such as a laser cavity, and a single solid-state laser system generally contains multiple isolators (anywhere from two to 10).
Traditional optical isolators are based on the Faraday effect, which is the rotation of a light beam’s plane of polarization caused by a magnetic field.
An isolator works by sending a light beam through a polarizer, which is angled to match polarization of the light. Then, the light passes through a transparent paramagnetic or ferrimagnetic material called a Faraday rotator, which rotates the polarization by 45 degrees. The light then passes through a second polarizer that also is angled at 45 degrees.
If any light reflects back, it is again rotated 45 degrees in the same direction by the Faraday rotator, so it reaches the first polarizer at an angle orthogonal to how it entered, preventing the light from passing through.

Currently, Faraday rotators are made using single crystals, typically terbium gallium garnet (Tb3Ga5O12, TGG). But could transparent ceramics be used instead?
That question interested ACerS Fellow Akio Ikesue, founder of specialty ceramics company World Lab. Co. Ikesue was the researcher who created the high-performing transparent laser ceramic in 1995, and so exploring whether transparent ceramics could be used in Faraday rotators naturally interested him as well.
His goal, however, was not just to create a polycrystalline version of TGG. He wanted to create a potentially better material.
TYO and TO ceramics: Improved rotator materials challenge conventional wisdom
Ikesue explains that in TGG, the Verdet constant—which indicates the ability of a material to polarize light—is determined dominantly by the occupancy of terbium ions. So, terbium-based materials that maximize terbium concentration and terbium ion occupancy would likely improve Faraday rotator performance.
In a paper published in 2017, Ikesue and his colleagues explain that various investigations revealed the most promising crystal structure to boost terbium ion occupancy is bixbyite (Re2O3, where Re is a lanthanide rare-earth element). However, using lanthanide rare-earth oxides as an optical material is, in a traditional view, inconceivable.
“The melting point of lanthanide rare-earth oxide is around 2,400°C, and it is very difficult to melt and solidify,” Ikesue explains. “Even if melting and solidification are possible, there is a phase transition point below the melting point in all lanthanide elements. Therefore, a large stress is induced in the material when it is produced, causing the material to break or crack. Also, a large stress associated with the phase transition remains inside the materials, and it cannot be used as an optical material.”
Ikesue says ceramic fabrication processes do not require melting, so there are less problems producing lanthanide rare-earth oxides this way than as single crystals. Unfortunately, high temperatures are still required to make these oxides fully dense and transparent, and the sintering temperature always exceeds the phase transition point around 1,500°C.
“Therefore, no one has ever succeeded in synthesizing this material even with ceramic technology,” Ikesue says.
Until recently, that is.
In the 2017 paper, Ikesue and his colleagues described their success creating terbium yttrium oxide (TYO) and binary terbium oxide (TO) ceramics using vacuum sintering and hot isostatic press treatment. They published another paper in 2019 improving on the process and describing TYO and TO properties, including the following.
High Verdet constants
The Verdet constants of (Tb0.6Y0.4)2O3 and Tb2O3 are 2.5 and 4 times that of TGG, respectively, meaning they can better polarize light. This fact means smaller magnets can be used to surround the Faraday rotator, thus encouraging device miniaturization.
High extinction ratio
In telecommunications, extinction ratio describes the efficiency with which transmitted optical power is modulated over fiber-optic transport.
Compared to the extinction ratio of TGG (35 dB), TYO and TO had extinction ratios of 42 dB and 47 dB, respectively, meaning they are more efficient at transmitting light.
Low insertion loss
Insertion loss describes the optical loss that occurs inside the Faraday rotator medium when polarized in a strong magnetic field.
TYO and TO showed comparable insertion loss to TGG.
In addition, TYO had a laser damage threshold of 18 J/cm2, which is about twice that of TGG single crystal, meaning TYO ceramics are less likely to suffer optical damage when used with a high-powered laser.

Ikesue says when they first began developing TYO and TO ceramics, they provided some Faraday rotator prototypes to their end users for evaluation. At the same time, they exhibited a very small and compact optical isolator device at the SPIE Photonics West conference in San Francisco. From these experiences, they found a large demand for the device, so they started selling it as a new product from the beginning of this year.
Ikesue says he and his colleagues succeeded in their research because of their willingness to look past conventional wisdom.
“We doubt past theoretical views and technologies and tried to know the truth of natural science with a pioneering spirit,” he says.
The 2017 paper, published in Optics Letters, is “Polycrystalline (TbXY1-X)2O3 Faraday rotator” (DOI: 10.1364/OL.42.004399)
The open-access 2019 paper, published in Materials, is “Total performance of magneto-optical ceramics with a Bixbyite structure” (DOI: 10.3390/ma12030421).