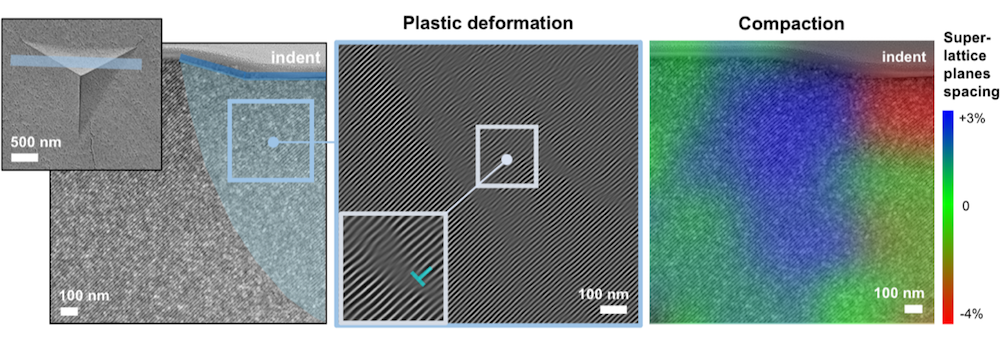
[Image above] Organically linked supercrystals are an emerging type of nanocomposite that could have uses in next-generation electronics and as biomimetic structural materials. The above images come from a recent paper exploring the deformation mechanisms of such materials. Credit: Giuntini et al., Science Advances (CC BY-NC 4.0)
When looking for a significant other, you likely have a checklist of characteristics that you wish they would fulfill. However, unless you are playing a dating sim, real-life relationships always involve tradeoffs, and deciding which characteristics are most important is key to a successful union.
The same concept applies to materials. When selecting a material for a certain application, you have a checklist of characteristics you wish for that material to fulfill. However, finding a material that fulfills every requirement is often difficult, so tradeoffs must be made to achieve an acceptable solution.
Fortunately for materials scientists, they have an advantage that wannabe suitors do not—they can take different materials that each have desirable properties and form composite materials to obtain combined properties not present in the single constituents.
In recent years, researchers have expressed particular interest in designing nanocomposites because materials can demonstrate significantly different properties on the nanoscale than in bulk form. For example, diamonds are known for being extremely hard and brittle, but on the nanoscale they can undergo full reversible elastic deformation at strain levels that would cause macroscopic diamonds to break.
Supercrystalline materials are one emerging approach to nanocomposite design. These materials consist of highly ordered, 3D assemblies of nanoparticles that are segmented into unit cells, i.e., the simplest repeating unit in a crystal, much larger than in ordinary inorganic crystals. Typically, the nanoparticles are assembled by interfacing with an ultrathin organic layer, which allows the composite to exhibit valuable inorganic and organic properties.
Thanks to this combination of nanosized building blocks and their periodic arrangement, supercrystals can feature a variety of functional properties, which give them great potential as structural bioinspired materials. In addition, supercrystals feature a quasi-isotropic mechanical behavior, meaning their mechanical properties have the same value when measured in different directions, in contrast to high-aspect-ratio layered structures.
Despite these advantages, researchers have struggled to fabricate organically linked supercrystals with good mechanical properties. That situation changed in 2016, when researchers published a letter in Nature Materials.
Led by Gerold Schneider, professor of materials mechanics at the Hamburg University of Technology in Germany, the researchers explored creating organically linked supercrystals using oleic acid and iron oxide nanoparticles.
They chose oleic acid as the organic linking molecule because it is a broadly used nanoparticle ligand, meaning any crosslinking approach developed with it should be applicable to a large variety of nanoparticle systems. They used iron oxide as the nanoparticle system because such nanoparticles can be synthesized with very good size control, and it also is producible in large amounts.
The researchers found they could create organically linked supercrystals with exceptional quasi-isotropic mechanical properties by adjusting the size of the nanoparticles.
“The main difference from the approaches used thus far is the adjusted size of the nanoparticles in a close-packed supercrystal, such that monolayers of oleic acid molecules on the surface of the nanoparticles can bridge the tetrahedral and octahedral sites,” they write. “This requirement is fulfilled for particle diameters smaller than 16 nm.”
When this bridging occurs, the weak van der Waals interactions that typically hold the unit cells together become overridden by a network of covalent bonds, resulting in a shift from soft matter to strong inorganic-organic nanocomposites.
The organically linked supercrystal demonstrated exceptional elastic modulus of up to about 60 GPa, bending strength of up to about 630 MPa, and hardness of up to about 4 GPa. “To our knowledge these are the highest combined values of elastic modulus, strength and nanohardness ever reported for a synthetic bioinspired organic/inorganic nanocomposite, and similar to microcantilever beam and micropillar strengths and moduli values of enamel,” the researchers write. (Since that study, the researchers increased these properties even more through further process optimization.)
Their success in achieving good mechanical properties for organically linked supercrystals is a significant milestone. However, as noted in the introduction, materials typically need to exhibit a variety of characteristics to fulfill a given application. Thus, understanding the other properties of a mechanically strong supercrystal is important for moving from lab to application.
In a new open-access study published this month, the researchers explored the deformation behavior of their organically linked supercrystal. They chose this property because, if the supercrystal proved to be both mechanically strong and deformable, it would be ideal for electronic applications, especially next-generation stretchable or bendable devices.
They performed nanoindentation experiments to measure elastic modulus and hardness of the organically linked supercrystals, and the measurements allowed them to subsequently assess the nanocomposite’s deformation behavior. In addition, they used a combination of atomic force microscopy, scanning electron microscopy, and transmission electron microscopy to visualize the supercrystalline structure, defects, and deformations.
Based on the experimental measurements, microscopy observations, and some accompanying finite element simulations, the researchers concluded that supercrystals, much like ordinary crystals, accommodate plastic deformation in the form of pileups, dislocations, and slip bands.
“The classic shear theories of crystalline materials are found to describe well the behavior of supercrystalline nanocomposites, which result to feature an elastoplastic behavior, accompanied by compaction,” they write.
Ultimately, the researchers believe the good mechanical properties and ability to deform plastically means organically linked supercrystals can find applications as robust components for batteries and sensors, as well as biomimetic structural materials and bioimplants. At this point, though, the supercrystals’ ductility and fracture toughness still need to be enhanced to reach the mechanical behavior desirable for flexible devices. But this study already unveils what can be achieved with crosslinked and noncrosslinked oligomers, and it defines the path toward further optimization.
In an email, first author and Eindhoven University of Technology assistant professor Diletta Giuntini says there are several plans for expanding on this research.
“I have received a grant from the German Research Foundation to explore defects, mechanical properties, and their correlations in supercrystalline nanocomposites. This is a fundamental study focused on the same material system, aimed at seeing how much the theories of the mechanical behavior of (atomic) crystalline materials are applicable/adaptable to supercrystals,” she says.
In addition, researchers in Hamburg University of Technology’s Collaborative Research Center SFB 986 plan to build supercrystalline nanocomposites with varying nanoparticle material, shape, size, and anchoring ligand, which will then be implemented into hierarchical materials with tailored mechanical behavior.
Finally, the researchers plan to collaborate with other experts to better understand how to control the particle–particle interactions in supercrystals, which will allow them to fine-tune mechanical behavior toward functional applications. “Here we will progressively move toward microparticles and larger organic molecules too. The goal is to facilitate their implementation into stretchable or bendable devices, for instance,” Giuntini says.
The 2016 paper, published in Nature Materials, is “Organically linked iron oxide nanoparticle supercrystals with exceptional isotropic mechanical properties” (DOI: 10.1038/nmat4553).
The 2021 open-access paper, published in Science Advances, is “Defects and plasticity in ultrastrong supercrystalline nanocomposites” (DOI: 10.1126/sciadv.abb6063).