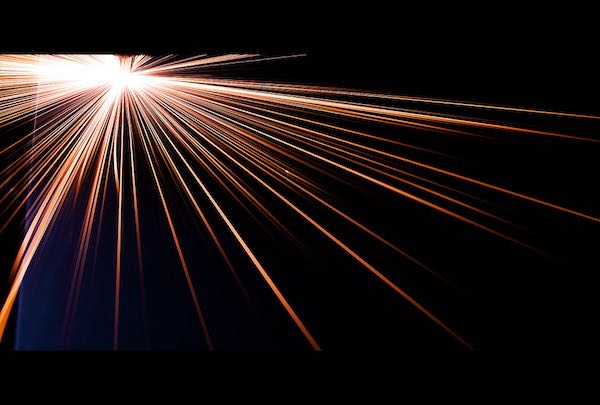
[Image above] Credit: Ilares Riolfi; Flickr CC BY 2.0
As cold and snow push into the United States this week and threaten to ice through next week, some of you might need something to warm you up.
Well, this news is, quite literally, hot.
Researchers at Imperial College London have discovered that hafnium carbide and tantalum carbide have some of the highest melting points of any measured materials—making these ultra-high temperature ceramics potentially perfect for use in extreme environments, such as on hypersonic vehicles that soar through space.
While scientists previously knew that these materials had high melting points—they’re called ultra-high temperature ceramics (UHTCs) for good reason—it’s not necessarily easy to heat and measure how materials melt at temperatures in the scorching vicinity of ~4,000ºC. And there are all sorts of other material challenges that arise at such high temperatures.
Previous information about the melting points of these materials was collected using less-than-precise methods during the mid-twentieth century.
We needed new technology to catch up—and lasers did the trick.
Using a laser melting technique, the Imperial researchers were able to more precisely measure the melting points of UHTCs tantalum carbide, hafnium carbide, and material mixtures of the two.
“For the first time in more than 50 years, the melting points of these materials have been reassessed experimentally,” Omar Cedillas-Barraza (now at University of Texas at El Paso), ACerS member and leading author on the Scientific Reports paper describing the research, writes in an email. ACerS member Michael Reece and ACerS president William Lee also are authors on the paper.
Cedillos-Barraza continues, “There has been discussion about the melting points of these materials for quite a while, so we used a novel laser heating technique, which has been tested with other high melting temperature materials of nuclear interest.”
Laser melting techniques have been used to measure other refractory materials including uranium carbides, uranium oxides, plutonium oxides, uranium nitrides, and zirconium carbides.
The technique works so well for high temperature assessments because the laser melts only localized areas of a sample at very short (subsecond) durations, bypassing some of the inherent materials challenges encountered at very high temperatures. The authors write in the paper that another advantage of this localized melting is that the non-melted portion of the sample forms its own “self-crucible,” preventing sample contamination.
While laser melting a mixed compound of Ta0.8Hf0.2C showed that its melting point was consistent with previous research (3,905°C), the melting points of individual samples of hafnium carbide or tantalum carbide were even higher than previous research suggested.
“We found that the highest melting point material is hafnium carbide, the highest ever measured for a material, and the highest in the tantalum carbide–hafnium carbide system,” Cedillos-Barraza writes. “The results of this research contradict previous results published related to these UHTCs, which claimed that tantalum carbide had a higher melting point and, in some papers, a solid solution of tantalum carbide–hafnium carbide had the highest melting point.”
The Imperial researchers measured a melting point for tantalum carbide of 3,768°C, while hafnium carbide had a record-breaking melting point of 3,958°C—the highest melting point measured for any material.
Such high melting points mean that these materials are refractory to heat even in extremely hot environments, so they could be particularly well-suited for applications in extreme environments, such as for thermal protection systems on hypersonic vehicles or nuclear fuel cladding.
Hypersonic vehicles can travel at speeds in excess of Mach 5. We’ve seen several recent developments at slower supersonic speeds (Mach 1–Mach 5), including commercial jets and record-breaking land vehicles. But even faster hypersonic speeds encounter even more heat, demanding materials that are that much more heat-resistant.
“The friction involved when travelling above Mach 5—hypersonic speeds—creates very high temperatures,” Cedillos-Barraza adds in Imperial College London press release. “So far, tantalum carbide and hafnium carbide have not been potential candidates for hypersonic aircraft, but our new findings show that they can withstand even more heat than we previously thought—more than any other compound known to man. This means that they could be useful materials for new types of spacecraft that can fly through the atmosphere like a plane, before reaching hypersonic speeds to shoot out into space. These materials may enable spacecraft to withstand the extreme heat generated from leaving and re-entering the atmosphere.”
Cedillos-Barraza continues, “Our tests demonstrate that these materials show real promise in the engineering of space vehicles of the future. Being able to withstand such extreme temperatures means that missions involving hypersonic spacecraft may one day be manned missions. For example, a flight from London to Sydney may take about 50 minutes at Mach 5, which could open a new world of commercial opportunities for countries around the world.”
While that prospect sounds tantalizing to anyone who’s spent hours on a long flight, it doesn’t mean hypersonic travel is within close reach—yet.
Cedillos-Barraza adds that there are other problems with these UHTC materials that need to be solved beyond their high melting points. “The main challenge right now is how we can improve the oxidation resistance of these materials so they can be used in very high temperature applications.”
Oxidation is such an aggravation—except in the bitter cold, where it can be some of the best chemistry imaginable.
In addition, the authors hint in the paper’s conclusion that there’s more work to be done, because higher melting points might still be awaiting discovery in other materials. “This should boost further experimental research on Ta-Hf-C-N compositions that might display even higher melting temperatures than those assessed for the ternary Ta-Hf-C system, in line with Hong and van de Walle’s theoretical predictions.”
The open-access paper, published in Scientific Reports, is “Investigating the highest melting temperature materials: A laser melting study of the TaC-HfC system” (DOI: 10.1038/srep37962).