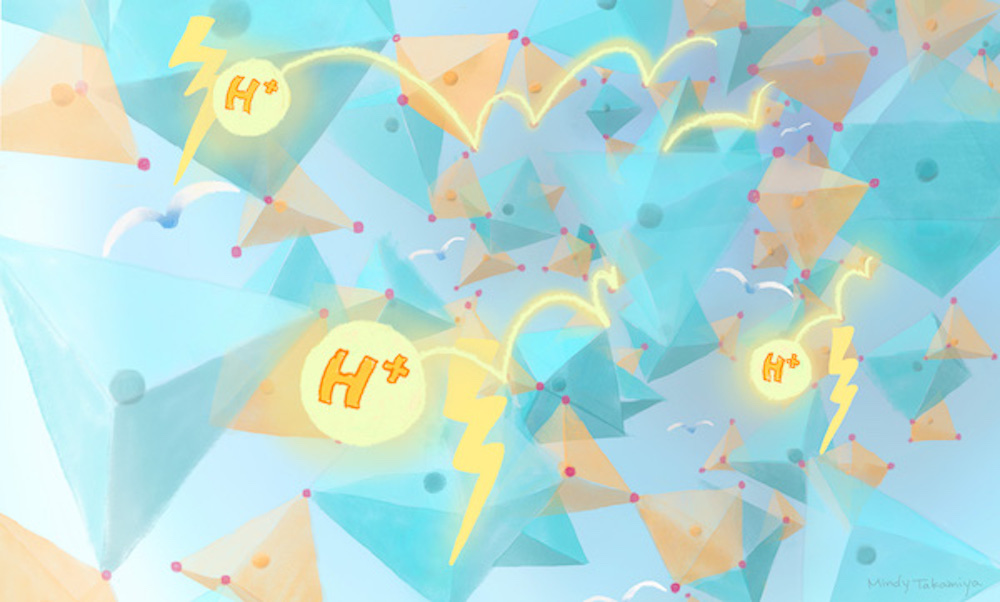
[Image above] The molecular structure of a new coordination polymer glass facilitates movement of protons across it under dry conditions at 120°C. Credit: Izumi Mindy Takamiya, Kyoto University (CC BY 4.0)
Nothing says the future of transportation quite like electricity.
In the past five years, the automotive industry has shifted markedly toward investing in electric cars. We first covered the shift on CTT in 2017, and investment has only continued to grow.
When people imagine electric vehicles, they generally picture a car containing a battery that must be recharged on a regular basis. Finding ways to hasten charging times and increase battery capacity are some of the main challenges associated with achieving an electrified future.
However, not all electric vehicles are battery powered. Another technology also being considered to power electric vehicles is fuel cells.
Fuel cells and batteries: What’s the difference?
Fuel cells are similar to batteries in that both produce an electric current through a chemical reaction. However, the mechanism by which they do so is slightly different.
To understand this difference, we need to look at the structure of these technologies. For both fuel cells and batteries, the basic setup consists of two electrodes (anode and cathode) on either end and an electrolyte (liquid or solid) in between.
In batteries, the system is self-contained—ions within the battery flow from the anode to the cathode upon discharge (and flow in reverse upon charging).
In fuel cells,* the system must get its molecules from an external source. Fuel, often hydrogen, supplies the anode with molecules. The molecules then pass over a catalyst and are separated, with the electrons sent through an external circuit to power the vehicle while the charged species (typically protons) flow through the electrolyte to the cathode, where they react with ambient air to produce water and heat.
In essence, while batteries store chemical energy for conversion into electricity, fuel cells generate energy only after an external source of fuel is supplied. The video below illustrates these differences quite well.
*Different types of fuel cells work in slightly different ways—for instance, solid oxide fuel cells do not require a precious metal catalyst. This description simply provides a general look at the technology.
PEM fuel cells: A leading contender
There are several types of fuel cells being developed, but a main contender for passenger vehicles is hydrogen-fueled polymer electrolyte membrane (PEM) fuel cells. Compared to other fuel cells, PEM fuel cells deliver high-power density with low weight and volume.
As the name suggests, the electrolyte layer of PEM fuel cells is based on a solid polymer membrane. However, for this membrane to work, it must be surrounded by liquid water.
“A dry membrane is a poor ionic conductor,” a Fuel Cell Store article explains. “As the dry [acidic] membrane absorbs water, it solvates the acid groups and becomes more conductive.”
But this water requirement places limitations on the fuel cell efficiency.
“The fuel cell efficiency increases at higher temperatures, but issues with membrane dehydration become worse, such as: reduction of ionic conductivity, decreased affinity for water, loss of mechanical strength via softening of the polymer backbone, and increased parasitic losses through high fuel permeation,” the Fuel Cell Store article says.
Thus, for PEM fuel cell researchers, developing proton conductive materials that do not require water to work well is a big goal—as evidenced by a recent open-access study.
Coordination polymer glass: A water-free electrolyte for PEM fuel cells
The researchers, from several universities, institutions, and corporations in Japan, explain that several studies in the past decade have focused on proton conductivity of coordination polymers (CPs) and metal–organic frameworks (MOFs).
“Crystalline CP/MOFs have advantages for high proton conductivity because of their tailorable pores and ability to accommodate guest molecules. However, they are intrinsically non-moldable because of their crystalline nature, and the grain boundary causes gas leaking and additional resistance in the electrolyte layers,” they write.
More recently, coordination polymers and metal–organic frameworks in liquid and glassy states have received attention as moldable materials. Despite their potential, “there are still a limited number of reports on proton-conductive CP/MOFs glass, and there are no reports of CP/MOFs to satisfy the criteria for sufficient proton conductivity (above 10 mS cm−1) under anhydrous conditions,” the researchers explain.
In their study, the researchers aimed to develop a CP glass with high intrinsic proton conductivity. They did so by focusing on protic ionic liquids.
Protic ionic liquids are a subset of ionic liquids (a salt in the liquid state) made by mixing an acid and a base. Some protic ionic liquid compositions have achieved high proton conductivity and high chemical and thermal stabilities, but they typically exhibit low viscosity, limiting use in membranes. Further, high mobility of both cations and anions in CP glass results in a low transport number of protons, which “leads to a decrease in the open-circuit voltage (OCV) in fuel cells,” the researchers write.
To overcome some of these problems, the researchers proposed using metal ions in addition to the protic ionic liquid when synthesizing CP glass.
“Appropriately selected metal ions can interact with the anions to form a CP with the desirable characteristics of moldability, proton conductivity, as well as a high transport number,” they write.
So they used zinc ions (Zn2+) and the protic ionic liquid diethylmethylammonium dihydrogen phosphate (dema)(H2PO4−) to synthesize a CP glass.
Structural analysis revealed large Zn–H2PO4−/H3PO4 coordination networks formed in the glass, which “results in enhancement of the properties of proton conductivity and viscoelasticity,” the researchers write. When tested in a hydrogen fuel cell, the glass produced a high open-circuit voltage of 0.96 volts, well within the range of current water-based electrolytes.

In a Kyoto University press release on the study, the researchers say they plan to continue their work with the aim of achieving fuel cell membranes with higher performance and long-term stability.
The open-access paper, published in Chemical Science, is “Coordination polymer glass from a protic ionic liquid: proton conductivity and mechanical properties as an electrolyte” (DOI: 10.1039/D0SC01737J).