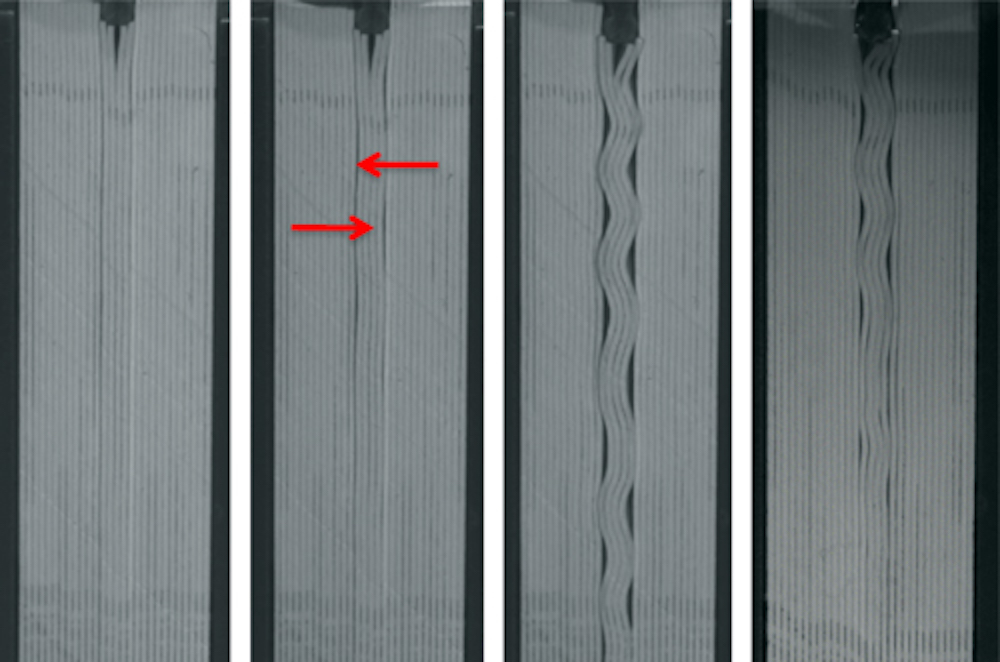
[Image above] Drexel University researchers demonstrated ripplocation deformation in macroscale objects, such as this deck of cards. They say a ripplocation framework can explain deformations in most layered solids. Credit: Michel Barsoum, Drexel University
To parody an old phrase, “there’s more than one way to deform an object.”
In January, we took a look at how glass deforms under volume and shear strains by modeling the response with a phase diagram. This month, we turn our study of deformations to a different state of matter: layered solids.
Layered solids are everywhere you look—from the subnanometer scale of graphene layers up to the 100-kilometer (and greater) range of geologic formations. Layered solids include a wide range of materials, from wood to mica to clay.
Because the term “layered solids” encompasses a vast size range and materials range, researchers commonly focus on studying specific subsets rather than similarities that apply to all layered solids. While this specialization makes tackling the task of understanding layered solids more manageable, it can result in mismatched ideas about layered solids when subsets are later compared.
An example of a mismatch is in the understanding of how layered solids deform. In layered crystalline solids such as graphite and mica, many scientists assume basal dislocations are the main cause of deformations. However, in all other domains of layered solids—including geology, wood, and layered composites—deformations are attributed to a buckling mechanism that forms kink boundaries.
This situation poses a question: are layered crystalline solids really different from all their fellow layered brethren, or is perhaps our understanding of layered crystalline solid deformation misguided?
ACerS Fellow Michel Barsoum and his colleagues at Drexel University and Colorado School of Mines posit support for the latter in a paper published last month.
Instead of basal dislocations, Barsoum and his colleagues argue that layered crystalline solids deform via nucleation and propagation of ripplocations, which are a “micromechanism by which layered solids deform,” Barsoum explains in an email. The term “ripplocations” (a word mashup of surface “ripples” and crystallographic “dislocations”) was coined by Akihito Kushima and co-authors in a 2015 paper, in which they used the term to describe near-surface deformations in 2D van der Waals solids.
Barsoum and his group were curious if this 2D phenomenon would apply to materials in bulk, so they applied a ripplocation framework to deformations occurring in graphite and MAX phase materials in three separate papers from 2016, 2017, and 2018. Conclusion: ripplocations describe bulk materials as well!
These first studies on ripplocations used simulations to model ripplocation deformations in materials. In the latest research, Barsoum and his group took ripplocations to the next level—by presenting direct macroscopic evidence for their existence.
“[This study] is the first time we can actually see ripplocations and [ripplocation boundaries] in action,” Barsoum says. They looked for ripplocations in three macroscale objects—a deck of plastic cards, thin steel, and aluminum sheets—and compared this deformation behavior to simulated models of ripplocations in graphite.
The researchers indented each stack of materials and found that once the indenter pressed the stacks to a critical indentation depth, a very rapid nucleation of multiple—and oppositely-signed—ripplocations occurred. Once the indenter was retracted, all layers spontaneously recovered to pre-pressed states.
“This first investigation showed that ripplocations exist and are more or less fully reversible and that they dissipate energy in a manner that we have observed in layered solids at the atomic scale for more than a decade now,” Barsoum says in a Drexel University press release. “But demonstrating the same behavior in layered materials that we can see directly is an important step toward proving that the behavior happens in materials of all sizes.”
In an email, Barsoum says this paper just “scratched the surface” of what can be studied about ripplocations, so it is too early to tell if all layered solids behave this way. “I leave the door open. I think that there [are] layered solids that are brittle, for example, and thus do not deform by the movement of ripplocations,” Barsoum explains. “The evidence so far on some of the newly discovered MAB phases appear that they microcrack rather than ripple. Also, in some cases, layered solids will twin and thus ripplocations do not play a role.”
In the future, Barsoum says there are a variety of other studies they can perform to build on this research. “There are a lot of variables that we need to test, like thickness of individual layers, friction between them, etc.”
The paper, published in Physical Review Materials, is “Ripplocations: A universal deformation mechanism in layered solids” (DOI: 10.1103/PhysRevMaterials.3.013602).